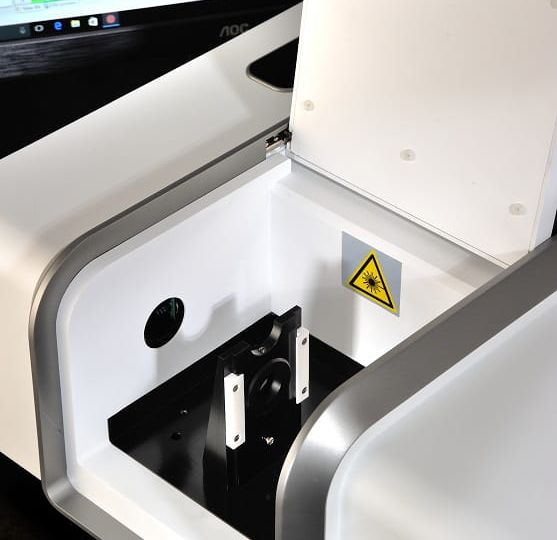
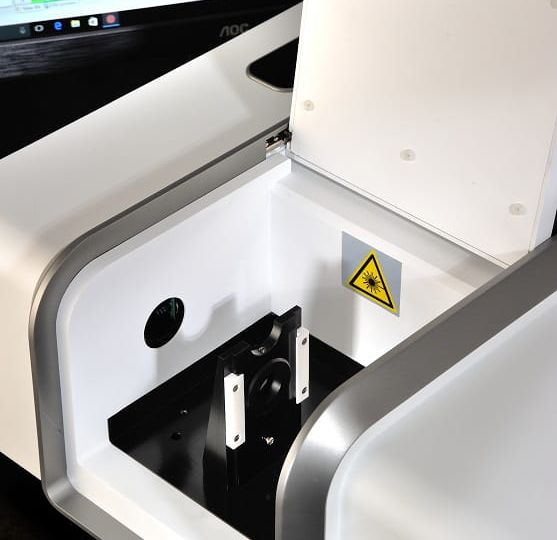
Introduction to Infrared Spectroscopy: Unlocking Molecular Secrets
Infrared and Fourier Transform Infrared (FTIR) Spectroscopy stand as the most prevalent spectroscopic techniques, measuring the absorption of various infrared frequencies by a sample exposed to an infrared ray. The primary objective of infrared spectroscopic analysis is to identify the chemical functional groups within the sample. Different chemical functional groups exhibit distinctive absorption frequencies of infrared radiation. With the aid of various sampling accessories, infrared spectrometers can analyze a wide range of sample types, including solids, gases, and liquids. Thus, infrared spectroscopy serves as a crucial and widely used tool for compound identification and structural analysis.
Historical Evolution of IR Spectroscopy
Since the 1940s, IR spectrometers have been commercially available. Initially, these instruments relied on prisms to act as dispersive elements. However, in the mid-1950s, diffraction gratings were introduced, enhancing the efficiency of these instruments. Significant advancements in IR spectroscopy emerged with the introduction of Fourier-transform spectrometers. These instruments, incorporating interferometers and utilizing Fourier transformation, have markedly improved data quality and minimized the time required for sample analysis. IR spectroscopy operates on the principle of molecular vibration. An IR spectrum is generated by passing IR radiation through a sample and assessing the fraction of incident radiation absorbed at specific energies. Each absorption peak in the spectrum corresponds to a vibration frequency of the sample molecule.
Understanding IR Frequency Range and Spectrum Presentation
The typical IR region spans from 4000 to 400 cm⁻¹. IR radiation encompasses wavelengths ranging from approximately 0.78 to 1000 μm, bordered by the red end of the visible region at high frequencies and the microwave region at low frequencies. IR absorption positions are typically presented using either wavelengths (λ) or wavenumbers (ν). Wavenumber, defined as the number of waves per unit length, is directly proportional to the frequency and energy of IR absorption. While wavenumbers (cm⁻¹) are commonly used in modern IR instruments, wavelengths are inversely proportional to frequencies. IR absorption information is typically presented in the form of a spectrum, with wavenumber or wavelength along the x-axis and absorption intensity or percent transmittance along the y-axis. Transmittance (T), the ratio of transmitted radiant power to incident radiant power, and absorbance (A), the logarithm of the reciprocal of transmittance, are commonly used measures. Transmittance spectra offer enhanced contrast between strong and weak bands, as transmittance ranges from 0% to 100%, while absorbance ranges from infinity (∞) to zero (0).
Selection Rule for IR Absorption
For a molecule to exhibit IR absorption, it must undergo a net change in the electric dipole moment during vibration. This change in dipole moment occurs as bonds within the molecule expand and contract. Additionally, the frequency of the radiation must match the natural frequency of vibration of the molecule for absorption to occur.
Theory of IR Absorption
At ordinary temperatures, atoms within molecules continuously vibrate with respect to each other. When IR radiation interacts with a molecule, it enhances these vibrations. Absorption of IR radiation by a molecule occurs when the frequency of a specific vibration matches the frequency of the incident IR radiation. Each atom in a molecule possesses three degrees of freedom, corresponding to motion along the three Cartesian coordinate axes (x, y, z). A polyatomic molecule comprising n atoms possesses a total of 3n degrees of freedom. However, three degrees of freedom describe translation (the motion of the entire molecule through space), and three describe rotation. Therefore, nonlinear molecules retain (3n – 6) fundamental vibrational modes, while linear molecules possess (3n – 5) fundamental vibrational modes due to fewer rotational degrees of freedom. Among these fundamental vibrations, those resulting in a net change in dipole moment may exhibit IR activity. The total number of observed absorption bands may differ from the total number of fundamental vibrations due to inactive modes and phenomena such as overtone and combination bands.
Modes of Vibration
The primary types of molecular vibrations are stretching and bending. Vibrations can involve changes in bond length (stretching) or changes in bond angle (bending).
Factors Affecting IR Spectra
Several factors can influence IR spectra, necessitating their consideration during spectral analysis to avoid misinterpretation of bands. These factors include combination tones, overtones, difference tones, vibrational coupling, Fermi resonance, and the physical state of the sample.
Fourier Transform Infrared (FTIR) Spectroscopy: Revolutionizing Analysis
FTIR spectroscopy relies on interference between two beams to produce an interferogram, which is then subjected to Fourier transformation to yield a spectrum. This technique offers numerous advantages, including faster data collection, enhanced sensitivity, mechanical simplicity, non-destructiveness, precision, and internal calibration. Applications of FTIR spectroscopy encompass the detection of impurities, reaction control, hydrogen bonding studies, configuration analysis, determination of bond distances, structural compound identification, assessment of sample quality or consistency, and quantification of components in mixtures.
Sampling Accessories
Modern FT-IR instruments employ various accessories to facilitate diverse analyses. These accessories include diffuse reflectance spectroscopy (DRS), photoacoustic spectroscopy (PAS), specular reflectance spectroscopy, emission spectroscopy (ES), and attenuated total reflectance spectroscopy (ATR).
Lambert–Beer Law
The Lambert-Beer law, formulated by August Beer in 1852, establishes a relationship between the absorption coefficient, concentration of the absorbing substance, and path length of light through the sample. This law serves as the foundation for infrared spectral analysis, enabling the quantification of absorbed radiation and subsequent determination of substance concentration.
Advantages of Fourier Transform Infrared (FTIR) Spectroscopy
FTIR spectroscopy offers several advantages, including improved speed, sensitivity, mechanical simplicity, non-destructiveness, precision, and internal calibration.
Applications of Fourier Transform Infrared (FTIR) Spectroscopy
FTIR spectroscopy finds application in a wide range of fields, including impurity detection, reaction control, hydrogen bonding studies, configuration analysis, determination of bond distances, identification of structural compounds, assessment of sample quality, and quantification of components in mixtures.
Conclusion
Infrared spectroscopy, particularly with the advancements in Fourier Transform Infrared (FTIR) technology, remains an indispensable tool in chemical analysis. Its ability to precisely identify and analyze a wide array of samples makes it invaluable in both research and industrial applications. By understanding and leveraging its principles, scientists and engineers can unlock deeper insights into the molecular world, driving innovation and discovery across various fields.
For more information, visit our FTIR product page. This content is optimized for SEO and contains internal links to ensure better visibility and navigation on your website:
- Learn more about Fourier Transform Infra-Red FTIR.
- Discover the Historical Evolution of IR Spectroscopy.
- Understand the IR Frequency Range and Spectrum Presentation.
- Explore the Theory of IR Absorption.
- Delve into the Modes of Vibration.
- Identify Factors Affecting IR Spectra.
- Gain insights into Sampling Accessories.
- Review the Advantages of FTIR Spectroscopy.
- Check out the Applications of FTIR Spectroscopy.
- Get detailed Specifications of FTIR Spectrometers.